Optical communications technology:
increasing capacity by more than 10,000 times over the last thirty years
—Mr. Miyamoto, what kinds of technical transitions have you seen in optical communications research to date?
First of all, let me point out that NTT has constructed a highly reliable and economical communications network that multiplexes and accommodates customer information from an access network like the telephone or fiber-to-the-home (FTTH) system, transmits this information via a high-capacity optical metro-core network using artery-like optical fibers, and distributes the information to specific areas, again by an access network. Since entering NTT, I have been engaged in the research and development (R&D) of ultrahigh-speed optical communications technology for high-capacity optical trunk-transport networks analogous to the shinkansen (bullet train) portions of the Japanese railway system.
In Japan, full-scale research on optical fiber as a transmission medium for optical communications began in the mid-1970s. At that time, progress in increasing the bitrate of the optical transmission system was made through optical fiber and electrical time division multiplexing (ETDM) based on integrated semiconductor circuits, and the first 32-Mbit/s commercial system was deployed in 1981.
I entered NTT in 1988, and about ten years later, the first single-mode optical fiber route along the length of Japan was completed and the first gigabit-class system, featuring a bitrate of 1.6 Gbit/s over a single optical fiber, was deployed. R&D of a next-generation 10-Gbit/s transmission system then commenced at NTT Laboratories and I was assigned to that project. Up to that time, optical communications had been progressing mainly as system technology for highly reliable and economical transmission of voice calls. This was an intensity-modulated direct-detection system that achieved high-capacity communications by high-speed on/off modulation of optical intensity using one wavelength (color). Here, a capacity of 10 Gbit/s was equal to about 130,000 telephone lines or the information requirements of one Japanese rural prefecture at that time, and this capacity was expected to be sufficient to meet future demands.
However, with the coming of the Internet in the latter half of the 1990s, the situation changed radically: it was recognized that data communications would become the dominant form of traffic on the network and that a dramatic increase in transmission capacity would be needed. Since then, optical communications R&D at NTT has brought forth a number of amazing system technologies, and today, transmission in excess of 1 Tbit/s over a single optical fiber can be achieved, which represents an increase from early bitrates of about 10,000 times.
—What is the reason for this dramatic increase in communication capacity?
Let me provide some background first. The intensity of an optical signal traveling through a transmission fiber is significantly attenuated after 100 km to as much as 1/100 to 1/1000 of the original level owing to optical-fiber loss. Thus, to maintain signal quality and achieve long-distance transmission, it was initially necessary to use optical repeaters, which convert light to electrical signals every 100 km as elements of repeater systems. However, to achieve even higher speeds with a practical intensity-modulated direct-detection system, determining how to significantly lower noise in this optical repeater became a major technical issue. In this regard, the development of the optical fiber amplifier in 1989 proved to be an epoch-making event.
The optical fiber amplifier amplifies light by using optical fiber with a core doped with rare-earth elements as an amplification medium. Despite its extremely simple structure, it can amplify input light with high efficiency, and since the amplification medium itself is optical fiber, it is highly compatible with optical fiber in the transmission path.
The erbium-doped optical fiber amplifier (EDFA) in particular can amplify light in the wavelength band where optical-fiber transmission loss is minimum (in the neighborhood of 1.55 µm). Furthermore, exciting it with short-wavelength light rather than signal light can cause energy to accumulate in the erbium, enabling the signal intensity to be increased very effectively. The EDFA can also transmit up to 100 channels (wavelengths) of information on a single optical fiber compared with only one wavelength in earlier optical communications systems. In short, the EDFA constitutes a wavelength-multiplexing system that can amplify multiple waves in a batch, enabling high-capacity transmission with very good efficiency.
The EDFA was first shown to be an effective device in 1989 and was first deployed about six years later. Its use suddenly lifted the transmission capacity of optical fiber into the 10-Gbit/s/wavelength region. Since then, systems using EDFA have undergone dramatic increases in transmission capacity as wavelength-division-multiplexing (WDM) systems that transmit information using multiple wavelengths. At present, EDFA systems with a transmission capacity of 1.6 Tbit/s per optical fiber are being put to practical use. This capacity corresponds to about 40 Gbit/s/wavelength, which is sufficient to transfer a two-hour DVD (digital versatile disc) movie in only 1 s.
As a key device that revolutionized information-communication networks, the EDFA¡½together with the Internet¡½has brought dramatic changes to the world. If EDFAs had never been developed, the advances that we have seen in broadband communications would probably have never materialized. This one innovative technology has not only altered research trends but also profoundly revolutionized the organizational structures of research laboratories around the world and the approach to system development (Fig. 1).
I witnessed this historical transformation brought about by the optical fiber amplifier only one year after joining NTT, and it was a revelation to me that a single technology could lead to such radical changes. Looking back, I was truly fortunate in being assigned to a project where NTT researchers worked diligently to research and develop innovative technologies and apply them to practical systems in a well-timed manner to bring something useful to society. This was a precious experience for me.
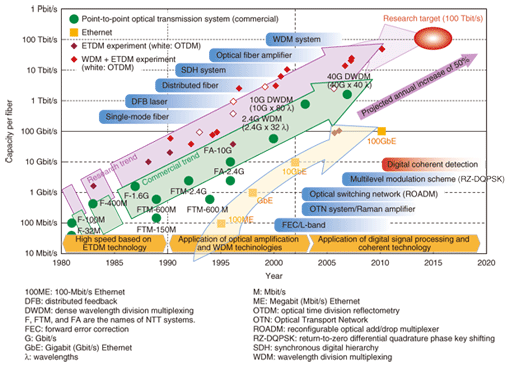
Fig. 1. Trends in optical transport technologies.
Ultrahigh-capacity digital coherent optical transmission technology: toward the 100-Tbit/s class
—Please tell us about your current research efforts.
If the current growth in data traffic continues unabated, a transmission capacity of the 100-Tbit/s class¡½or 100 times the current level¡½will be needed ten years from now. With this in mind, I¡Çm looking to develop optical communications system technologies to support this new era in communications. This will require the development of new technologies in addition to enhancing the performance of existing technologies. In this regard, I have high expectations for ultrahigh-capacity digital coherent optical transmission technology.
Digital coherent optical transmission technology combines optical coherent transmission technology and digital signal processing. Coherent technology achieves high-capacity transmission by using not only the intensity but also the frequency, phase, and polarization of the carrier wave used to transmit information. As in wireless technologies like mobile communications and wireless local area networks, combining coherent technology with digital signal processing can produce a jump in transmission speed and capacity.
The current high-capacity optical communications system has a transmission capacity of more than 1 Tbit/s on a single optical fiber using multiple wavelengths. Nevertheless, the range of wavelengths that an optical amplifier or repeater can handle at one time is limited and is currently equal to a bandwidth of about 4 THz. Thus, to achieve even higher capacities, we need technology that can pack even more signals into a single optical fiber. The answer is digital coherent transmission technology, which applies and expands upon signal-processing techniques used in wireless communications to raise the transmission capacity. Specifically, this technology simultaneously modulates optical intensity and phase to increase transmission capacity per wavelength in a limited frequency band and therefore improve spectrum efficiency.
In the beginning, it was thought that the application of digital coherent transmission technology could increase transmission capacity by 10 to 100 times that of current levels. This would require, however, that the throughput of the semiconductors (LSIs) used for signal processing be greater than 1 Tbit/s. Achieving such ultrahigh-speed signal processing has traditionally been difficult, but recent advances in semiconductor technology are now making it possible. At present, digital coherent transmission technology can be used to achieve stable transmission at a capacity of 100 Gbit/s per wavelength and an overall transmission capacity of about 10 Tbit/s per optical fiber. This technology is therefore maturing and approaching a practical level.
In addition, it appears that the recent development of a new technology has increased the above transmission speed by ten times in laboratory experiments, thereby bringing transmission capacity up to the 100-Tbit/s class. At NTT Laboratories, it has been about five years since we began R&D on 100-Gbit/s-class system technology, and throughout this time, our papers have regularly been selected at leading international conferences in post-deadline sessions where the latest research results compete with each other. In this way, we have been developing technology by competing head-on with other top transmission performance results (Fig. 2). Competition of this kind at international forums has helped us to accelerate our R&D efforts while making sure that our technology is always moving in the right direction in terms of global trends in communications system development.
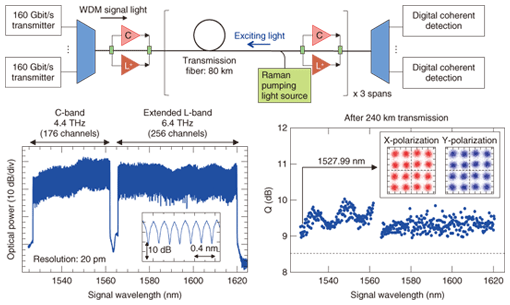
Fig. 2. 69.1-Tbit/s optical transmission experiment using polarization-division-multiplexing 16QAM (quadrature amplitude modulation) digital coherent optical modulation/demodulation technology [1].
Pampered generation of Japan: the electronics nation
—Your original research target was semiconductors, wasn¡Çt it? What led you to research optical fiber?
I entered NTT at the height of Japan¡Çs economic bubble, and during that time, people of my age group were called the pampered generation. But this pampering could not go on forever, and in time, the bubble burst, bringing an economic slowdown to Japan centered on the financial industry. Many of my fellow university students had planned to look for work in the financial industry, and several of my friends had a hard time because of this drastic change in the economy. I myself liked to make things from an early age, and as I wanted to pursue hardware-related work, I majored in fundamental semiconductor research at university. Then, seeking a field useful to society that would apply semiconductors and challenge me at the same time, I entered NTT. At that time, Japan, which was taking the world by storm with semiconductor technologies, was often called the electronics nation, and it was naturally expected that it would continue to develop in that direction. During that era, many NTT researchers were doing excellent work in the field of ultrahigh-speed semiconductors, and that was also a factor in my entering NTT.
At NTT Laboratories, I was assigned not to a department that researched semiconductors themselves but to one that researched high-capacity optical communications systems making use of semiconductors. Although I had first heard about the field of optical communications systems from lectures in graduate school and found it somewhat interesting, semiconductors was still the only field I knew anything about up to then. In other words, the field of optical communications systems was a completely unknown world to me at that time. To make matters worse, my knowledge of light and optics was minimal upon entering the company, and I really wondered whether I could survive in this field. This was such a source of anxiety for me that I sometimes thought about quitting (laughs).
However, not long after I entered NTT, the revolutionary EDFA technology that I mentioned earlier began to recast the world of peripheral platform technologies and optical communications systems, and I was fortunate to become part of this process. Looking back to that time, I remember getting a circuit that I designed myself to run in a stable manner, enabling me to contribute, even if only slightly, to the R&D process. This experience and the feeling of accomplishment that it engendered provided me with an important foundation for my future R&D work (Photo 1). Later, during difficult times, I was able to continue with my research without giving up, perhaps because I also had a number of experiences of being part of a wave of major technical innovations at that time. I am also happy to see that today¡Çs digital coherent technology is also unfolding in conjunction with semiconductor technology.
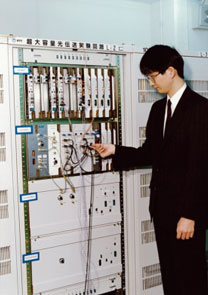
Photo 1. Mr. Miyamoto performs a field trial on a 10-Gbit/s optical amplifier system that originated in Tokyo not long after he joined NTT.
—Mr. Miyamoto, could you leave us with some advice for young researchers?
I would be happy to. First, in research fields involved in systems R&D, it is very important that researchers pursue their work while imagining scenes in which technology provides something useful for society. The R&D work that we are currently pursuing ranks as an advanced field in optical communications, but it is important to achieve a balance here between fundamental and applied research so that this field does not simply become research for its own sake but produces technology with practical applications. If it shows absolutely no affinity with existing technologies, even a long-awaited technology may be unable to demonstrate its value. With this in mind, I believe that the ideal way of researching advanced technologies is to keep involved with technical issues in the real world. This is the first step toward well-timed application of advanced technology. I believe that making a contribution to society through research results that can be used in practice cannot help but make researchers feel that their work has been worthwhile.
Second, I would like young researchers to keep in mind that today¡Çs research achievements build upon the proven results achieved by our predecessors and benefit from the development of peripheral technologies. Of course, results achieved through individual efforts are also essential, but not all advanced technologies will make it simply by virtue of being advanced. In this industry, going from nothing at all to a highly competitive product is a most difficult task.
In fact, a coherent detection scheme for configuring a digital coherent system was being vigorously researched twenty years before I arrived at NTT, and this coherent detection technology was slated to be an alternative to the intensity-modulated (on/off) direct-detection system used at that time and the next big thing in optical communications technology toward ultrahigh-speed, high-capacity transmission. However, the appearance of the EDFA in 1989 dramatically raised the performance of intensity-modulated (on/off) direct detection, and the coherent technology that had been progressing up until then was put on the back-burner where it has been for about twenty years. Now, however, developments in digital signal processing technology are helping coherent technology to make a strong comeback after more than twenty years, though in a somewhat different form.
If there had been no research foundation left to us by our seniors who developed this coherent detection system, it would no doubt have been difficult for us to achieve the present WDM transmission system and the recent digital coherent system. Furthermore, these systems depend on basic technologies like high-performance semiconductor lasers and optical passive devices, and if those technologies had not been established, today¡Çs advanced results might have been difficult to obtain. What this all means is that we engage in R&D in a rich, privileged environment founded on past achievements. I would therefore like young researchers to be aware of this and appreciate it and use this knowledge to good effect in future research.
These things, however, are difficult to understand simply on the basis of a verbal explanation. Thus, as I once learned from my superiors, I would like to impress upon young researchers the importance of creating an environment that can provide hints toward the next phase of R&D. This can be accomplished by identifying the technical problems involved in the practical application of technology and participating in the process of solving those problems.
Reference
[1] |
T. Kobayashi, S. Yamanaka, H. Kawakami, S. Yamamoto, A. Sano, H. Kubota, A. Matsuura, E. Yamazaki, M. Ishikawa, K. Ishihara, T. Sakano, E. Yoshida, Y. Miyamoto, M. Tomizawa, and S. Matsuoka, ¡È8-Tb/s (80¡ß127Gb/s) DP-QPSK L-band DWDM Transmission over 457-km Installed DSF Links with EDFA-only Amplification,¡É OECC2010, Sapporo, Japan, July 2010. |
↑ TOP