Feature Articles: Healthcare Devices and Infrastructure-maintenance Technology that Support People and Society
Vol. 19, No. 6, pp. 48–54, June 2021. https://doi.org/10.53829/ntr202106fa7
Physical-condition Management Technology for Creating More Comfortable Work Sites
Kazuhiko Takagahara, Yuki Hashimoto, Kenichi Matsunaga,
Yuichi Higuchi, Nobuaki Matsuura, Kei Kuwabara,
Hiroyoshi Togo, Takashi Kawahara, Akimasa Hirata,
Hideto Tanaka, and Taiki Miyazawa
Abstract
Physical-condition management is becoming more important to improve the safety of work sites, such as preventing heat stroke caused by intense heat. We at NTT Device Innovation Center have developed a physical-condition management technology for creating more comfortable work sites by using the wearable-device sensors and data-analysis technology we have developed thus far, and the knowledge of thermal and exercise physiology in collaboration with specialists. This article introduces the problems with physical-condition management, how our technology can solve these problems, and the results from verifying our technology at an actual work site.
Keywords: wearable sensor, physical-condition management, heat countermeasures
1. Introduction
The number of emergency transports and deaths due to heat stroke has increased, and reducing them has become a major social issue in Japan. According to a survey by the Fire and Disaster Management Agency, 64,770 people were transported by emergency due to heat stroke from June 1st to October 4th, 2020. According to the Ministry of Health, Labor and Welfare (MHLW), there were 829 casualties (death and leave of absence 4 days or more) due to heat stroke in the workplace in 2019, of which 25 died. MHLW has published guidelines and manuals for heat-stroke prevention measures in the workplace [1]. We at NTT Device Innovation Center have been engaged in research and development of physical-condition management technology using wearable biological/environmental sensors to solve such social issues and create more comfortable work sites.
2. Technical problems
We developed our physical-condition management technology to make work sites more comfortable from two aspects: real-time control of the risk of poor physical condition due to heat and reduction of accident risk by short- and medium-term operation management.
Figure 1 shows a schematic of the cause of poor physical condition due to activities in a hot environment [2]. When the body temperature rises due to exercise in normal environments, the heat is dissipated to the outside air due to sweating and increase in skin temperature, regulating body temperature. However, in a hot environment, it is difficult for heat to dissipate to the outside air. If the water and salt lost due to sweating are not sufficiently replenished, blood flow will also be impaired and heat will easily accumulate in the body, causing poor physical condition.
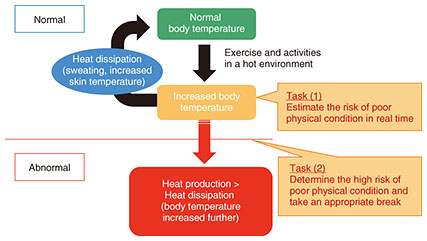
Fig. 1. Cause of poor physical condition in a hot environment.
We have found two requirements to mitigate such conditions. One is that it is necessary to estimate the risk of poor physical condition for each individual in real time because the environment, such as outside temperature and humidity at the work site, and the intensity of work and exercise vary from person to person and change from moment to moment. The other is that it is necessary to determine the high risk of poor physical condition from the estimation results to properly encourage breaks and water intake before poor physical condition occurs.
To reduce accident risk by short- and medium-term operation management, we applied the acute chronic workload ratio (ACWR), which is attracting attention as being effective in reducing the risk of injury and poor physical condition of athletes, to workers. The ratio of short-term work load of about one week to long-term work load of about one month is defined as the ACWR. The risk of injury increases if the ACWR is too high or too low, so daily operation is managed so that the ACWR is in the low risk area. One of the problems is quantifying the work load caused by daily work.
To solve these problems, we developed a wearable sensor for acquiring biological/environmental information and a sensor-data-analysis technology based on thermal and exercise physiology.
3. Key technologies
3.1 Wearable biological/environmental sensor
To estimate the risk of poor physical condition for each worker in real time, it is necessary to acquire various biological and environmental information without interfering with work, and we believe wearable sensors are effective for this purpose.
Figure 2 shows our wearable biological/environmental sensor. It consists of a transmitter (TX02), which is commercialized by NTT TechnoCross based on the wearable biological/environmental sensor technology researched and developed at NTT Device Innovation Center [4], a hitoeTM*1 shirt, hitoe belt from Toray Industries, Inc., or C3fit IN-pulse*2 shirt from GOLDWIN INC. Figure 3 shows a schematic of data acquisition. The user wears the garment as underwear so that the hitoe electrode is in direct contact with the skin, and the TX02 is attached to the garment via the connector. The weak bioelectric signal generated from heart beats is acquired from the hitoe electrode, input to the TX02 via the connector, and recorded as electrocardiographic data by the bioelectric signal sensor. In addition to the bioelectric signal sensor, the TX02 has a built-in temperature/humidity sensor and acceleration/angular velocity sensor. Therefore, it is possible to measure data on an electrocardiogram, temperature and humidity inside clothes, and acceleration and angular velocity of the upper body. Various features such as heart rate, heart-rate interval, number of steps, and upper body tilt, are also analyzed from the measured data.
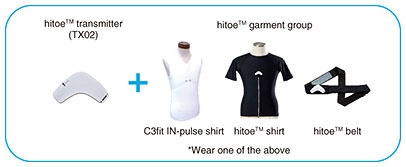
Fig. 2. Wearable biological/environmental sensor.
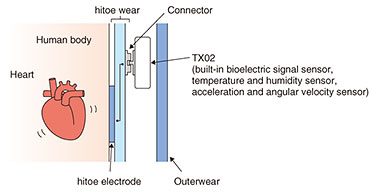
Fig. 3. Schematic of data acquisition.
3.2 Core-temperature-fluctuation estimation
Body-core temperature*3, heart rate, and subjective symptoms of dizziness and nausea are listed as indicators of poor physical condition in hot environments [5]. Conventionally, the body-core temperature is measured by inserting a thermometer into the esophagus or rectum, which requires specialized skills, and is difficult to measure it in real time during activities such as work and exercise.
Therefore, we developed a technology for estimating core-temperature fluctuations in real time during activity through joint research with Nagoya Institute of Technology. With this technology, body-temperature fluctuation is estimated by inputting the information acquired from the wearable sensor into a newly constructed calculation model. The overview of this model is shown in Fig. 4. The human body is divided into a core layer and skin layer, and the heat exchange between each layer and the outside air is formulated. The formula uses an algorithm developed by Nagoya Institute of Technology that takes into account factors such as heat production from human activities, thermoregulatory function due to sweating etc., and clothing. Heart rate, temperature/humidity inside clothes, and personal information (age, height, weight, gender, clothes, etc.) are input to this model to calculate fluctuations in core temperature. With this model, it has become possible to calculate core-temperature fluctuations in real time even with the processing power of smartphones.
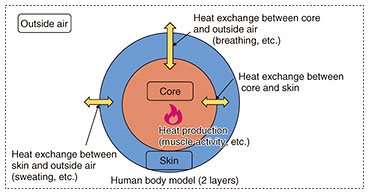
Fig. 4. Calculation model of core-temperature fluctuation.
To confirm the usefulness of this estimation technology, we conducted a joint clinical experiment*4 with NTT, Yokohama National University, Shigakkan University, and Nagoya Institute of Technology. This experiment was conducted in the artificial weather room of Shigakkan University to simultaneously estimate body-temperature fluctuations using this technology and with a rectal thermometer. We confirmed that the estimation accuracy of our technology was sufficient to determine the risk of poor physical condition.
3.3 Determine risk of poor physical condition
Indicator criteria and algorithms are required to determine the high risk of poor physical condition for each worker. On the basis of the knowledge obtained from the joint experiment and from thermal physiology, we set criteria for three indicators: heart rate, estimated core-temperature fluctuation, and subjective information. We also constructed an algorithm that determines the overall risk of poor physical condition from the state of the three indicators and issues an alert in the case of high risk.
3.4 Work-load estimation
To quantify the workload caused by daily work, it was conventionally necessary to obtain the rate of perceived exertion from questionnaires as soon as possible after the work. We developed a technique to estimate the rate of perceived exertion through heart-rate analysis based on exercise physiology without using a questionnaire and confirmed its effectiveness with the cooperation of the Waseda University Rugby Club [6].
*1 |
hitoeTM: The functional fabric hitoe is a fiber material jointly developed by Toray Industries, Inc. and Nippon Telegraph and Telephone Corporation, and is a trademark of the two companies. |
*2 |
C3fit IN-pulse: A clothing line that monitors physiological information using hitoe offered by GOLDWIN INC. |
*3 |
Body-core temperature: Temperature inside the head or trunk. |
*4 |
Shigakkan University Research Ethics Review Committee No. 124. |
4. Verification in the workplace
To verify the usefulness of our physical-condition-management technology at work sites, we conducted a verification experiment*5 targeting construction workers in the Tokyo, Kanagawa, and Hokkaido regions from August to September 2020 with the cooperation of NTT EAST. Figure 5 shows a schematic diagram of this experiment. We implemented our core-temperature-fluctuation-estimation technology, algorithm for determining the risk of poor-physical condition, and work-load-estimation technique as smartphone applications and built a demo system that remotely monitors the physical condition of each worker wearing wearable biological/environmental sensors via the cloud (this demo system is not for medical use). Alerts were sent to workers who were determined to be at high risk.
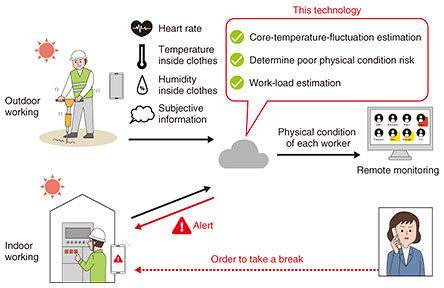
Fig. 5. Schematic of verification experiment at the work site.
To confirm the usefulness of the core-temperature-fluctuation-estimation technology and work-load-estimation technique at the work site, we evaluated the relationship between the subjective information (warm/cold feeling, rate of perceived exertion) obtained from the workers and the estimation results. We confirmed that there is a correlation between the estimated core-temperature fluctuation and warm/cold feeling and between the estimated work load and the rate of perceived exertion. These results indicate the possibility of visualizing the subjectivity of workers without using questionnaires and the usefulness of core-temperature fluctuation estimation and work-load estimation based on wearable biological/environmental sensor data.
Risk cases were comprehensively detected during the verification by the three indicators and the algorithm for determining the risk of poor physical condition. Alerts were sent to remotely monitoring managers and workers. Since no serious cases of poor physical condition, such as heat stroke, occurred during this verification experiment, we consider our technology useful.
*5 |
The Japanese Society for Wellbeing Science and Assistive Ethics Review Committee No. 286. |
5. Summary
To make work sites more comfortable, we developed a physical-condition-management technology that involves wearable sensors and verified it at a work site. We will continue to deepen our knowledge by introducing our technology at more work sites and promote further improvements.
References
[1] |
Ministry of Health, Labor and Welfare, “Heat Stroke Prevention Measures in Workplace” (in Japanese),
https://www.mhlw.go.jp/stf/seisakunitsuite/bunya/0000116133.html |
[2] |
Ministry of the Environment, Heat Illness Prevention Information, Heat illness prevention/countermeasures,
https://www.wbgt.env.go.jp/en/doc_prevention.php |
[3] |
T. J. Gabbett, “The Training-injury Paradox: Should Athletes Be Training Smarter and Harder?”, Br. J. Sports Med., Vol. 50, No. 5, pp. 273–280, 2016. |
[4] |
Focus on the News: “Development of Low-power and Compact Wearable Sensor for Measuring Electrocardiographic, Acceleration, Temperature/Humidity Data for Use in Smart Healthcare,” NTT Technical Journal, Vol. 32, No. 3, pp. 57–58, 2020 (in Japanese). |
[5] |
American Conference of Governmental Industrial Hygienists, “Threshold Limit Values and Biological Exposure Indices,” 2019. |
[6] |
Focus on the News: “Development of Technology for Supporting Scientific Training in Sports and Demonstration of the Feasibility for Rugby Players,” NTT Technical Journal, Vol. 32, No. 7, pp. 60–62, 2020 (in Japanese). |
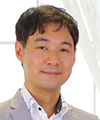 |
- Kazuhiko Takagahara
- Senior Research Engineer, NTT Device Innovation Center.
He received a B.S. in mechanical engineering and M.S. in information science and technology from the University of Tokyo in 2006 and 2008. He joined NTT Microsystem Integration Laboratories in 2008, where he has been engaged in research and development (R&D) of optical and wireless telecommunication devices and vital sensing devices. He is a member of the Japan Society of Applied Physics.
|
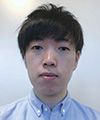 |
- Yuki Hashimoto
- Engineer, NTT Device Innovation Center.
He received a B.E., M.E., and Ph.D. in engineering from Tokyo Institute of Technology in 2014, 2016, and 2018. He joined NTT Device Innovation Center in 2018 and is currently researching wearable healthcare devices for management of heat stress. He is a member of the Japan Society of Mechanical Engineers and the Institute of Electrical Engineers of Japan.
|
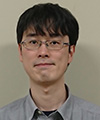 |
- Kenichi Matsunaga
- Research Engineer, NTT Device Innovation Center.
He received an M.E. in electronics from Tokyo Institute of Technology in 2010 and joined NTT Microsystem Integration Laboratories the same year, where he studied low-power complementary metal oxide semiconductor (CMOS) circuits design for wireless sensor nodes. Since 2015, he has been with NTT Device Innovation Center, where he is currently developing Internet of Things devices. His research interests include CMOS circuits and sensor networks. He is a member of the Institute of Electronics, Information and Communication Engineers (IEICE) and the Institute of Electrical and Electronics Engineers (IEEE).
|
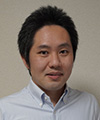 |
- Yuichi Higuchi
- Manager, Digital Twin Business Department, NTT TechnoCross Corporation.
He received a B.E. and M.E. from Kyoto University in 2006 and 2008. He joined NTT Microsystem Integration Laboratories in 2008, where he was engaged in R&D of optical telecommunication devices and vital sensing devices. In 2020, he moved to NTT TechnoCross, where he is currently in charge of the healthcare service development. He is a member of IEICE and the Japan Society for Precision Engineering.
|
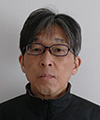 |
- Nobuaki Matsuura
- Senior Research Engineer, NTT Device Innovation Center.
He received a B.S. in applied physics and M.E. in energy science from Tokyo Institute of Technology in 1990 and 1992. He joined NTT in 1992 and has been conducting research on optical interconnection technology. He is a member of IEICE.
|
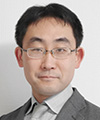 |
- Kei Kuwabara
- Senior Research Engineer, Project Planning Section, NTT Device Innovation Center.
He received a B.E. and M.E. in electronics engineering from Keio University, Kanagawa, in 2002 and 2004. He joined NTT Microsystem Integration Laboratories in 2004 and studied micro electro-mechanical systems for wireless and optical networks. Since 2013, he has been engaged in R&D of wearable devices for healthcare, such as wearable blood-flow sensors and hitoe, and in 2015, was responsible for promoting open collaboration of hitoe in the R&D planning department. He is currently in charge of promoting R&D of NTT Device Innovation Center. He is a member of IEEE and IEICE.
|
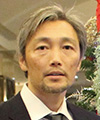 |
- Hiroyoshi Togo
- Senior Research Engineer, Supervisor, NTT Device Innovation Center.
He received an M.Sc. and Ph.D. in applied physics and electronic engineering from the University of Tsukuba, Ibaraki, in 1996 and 2010. He joined NTT Musashino Opto-electronics Laboratories in 1996. Until 2001, he was involved in the development of the thermo-capillary waveguide-based optical switch and from 2001 to 2002 endeavored to commercialize it for NTT Electronics. From 2003 to 2015, he developed ultrawideband impulse radio systems using photonic techniques and millimeter-wave tomography with electro-optic probing at NTT Device Technology Laboratories. Since 2016, he has been leading a wearable-device development project to contribute to smart healthcare at NTT Device Innovation Center. He is a senior member of the IEEE Photonics, Antenna and Propagation, and Microwave Theory and Techniques Society, and IEICE of Japan. He was a finance director of the Council of Technical Committee Representatives and a director of the Technical Committee on Photonics-applied Electromagnetic Measurement in the IEICE Communications Society. He is also an expert member of the Technical Committee on Microwave Photonics in the IEICE Electronics Society and an expert member of the Japan Electronics and Information Technology Industries Association wearable electronics standardization. He received the 2006 Asia Pacific Microwave Photonics Conference AP-MWP Award, the 2010 European Conference on Antenna and Propagation Award, and The Japan Wood Research Society Best Paper Award 2013.
|
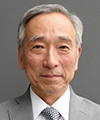 |
- Takashi Kawahara
- Research Professor, NTT.
He graduated Medical School, the University of Tokyo in 1976. After his medical internship, he specialized in cardiology and worked at the Hospital, the University of Tokyo. He became lecturer at the Department of Physical Education and Sport Sciences, College of Arts and Sciences, the University of Tokyo in 1985, associate professor in 1989. He became director of Sport Medicine, Japan Institute of Sport Sciences in 2001, general director of Japan Institute of Sport Sciences in 2014, and retired in 2017. He joined NTT as research professor in 2019. His main research areas were prevention of disorders caused by sport (such as heat stroke, overtraining syndrome, anemia) and hypoxic training. He participated in the Olympic Games six times as the Japanese delegation doctor. He is currently vice president of the Japan Association for University Athletics and Sport, chairman of Sport Medicine and Science Commission, Japan Sport Association.
|
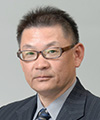 |
- Akimasa Hirata
- Professor and Research Director, Nagoya Institute of Technology.
He received a B.E., M.E., and Ph.D. in communications engineering from Osaka University in 1996, 1998, and 2000. From 1999 to 2001, he was a research fellow of the Japan Society for the Promotion of Science (JSPS) and a visiting research scientist at the University of Victoria, Canada, in 2000. In 2001, he joined the Department of Communications Engineering, Osaka University, as an assistant professor. In 2004, he joined the Department of Computer Science and Engineering, Nagoya Institute of Technology as an associate professor where he is currently a full professor. His research interests include electromagnetic safety, risk management system for heat-related illness, methods in neuroscience, antennas, and related computational techniques. He is an editorial board member of Physics in Medicine and Biology, a member of the main commission and a chair of project group of International Commission on Non-Ionizing Radiation Protection, a member of administrative committee and a subcommittee (EMF Dosimetry Modeling) chair of IEEE International Committee on Electromagnetic Safety, and an expert of the World Health Organization. He received several awards including Young Scientists’ Prize (2006) and Prizes for Science and Technology (Research Category 2011, Public Understanding Promotion Category 2014, 2020) by the Commendation for Science and Technology by the Minister of Education, Culture, Sports, Science, and Technology, Japan, and IEEE EMC-S Technical Achievement Award (2015), and the Japan Academy Medal and JSPS Prize (2018). He is a fellow of IEEE, Institute of Physics, and IEICE.
|
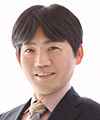 |
- Hideto Tanaka
- Professor, Graduate School of Education, Yokohama National University.
He received a Bachelors of Health and Sports Science and an M.A. in health education from the University of Tsukuba, Ibaraki, in 1981 and 1983 and a Ph.D. in medical science from Osaka University in 1988. He has been an assistant at the School of Medicine, Osaka University since 1983, conducting research on thermal physiology, especially thermoregulation during exercise. He then became a lecturer at the Faculty of Education, Yokohama National University in 1990, associate professor in 1991, and professor in 2004. From 1994 to 1995, he was appointed as a visiting researcher at the Faculty of Psychology, University of Delaware, USA. His research areas are exercise physiology and environmental physiology, with a focus on thermal physiology. His current main research themes are thermoregulation during exercise, the living environment and thermoregulatory function, and prevention of heat stroke. In 2002, he received the 5th Chichibunomiya Memorial Sports Medicine and Science Encouragement Award for “Study of Heat Stroke Prevention (Principal Investigator: Dr. Kawahara).” His main academic societies are the Japanese Society of Physical Fitness and Medicine, the Japan Society of Exercise Physiology, the Japanese Society of Biometeorology, and the Physiological Society of Japan.
|
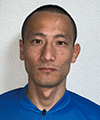 |
- Taiki Miyazawa
- Associate Professor, Department of Health and Sports Science, Faculty of Wellness, Shigakkan University.
He received a B.E. in mechanical engineering from Osaka City University in 2004, M.E. in physics system of machine from the Graduate School of Engineering, Osaka City University, in 2006, and Ph.D. in medical science from the Graduate School of Medicine, Osaka City University, in 2010. He was a postdoctoral fellow with the University of Toyo, Saitama, from 2010 to 2012, the National Institute of Advanced Industrial Science and Technology, Ibaraki, from 2012 to 2013, and Kyorin University, Tokyo, from 2013 to 2015. He is currently an associate professor with Shigakkan University, Aichi. His current research interests include body-temperature regulation and cerebral blood-flow regulation during exercise.
|
↑ TOP
|